Introduction
In the expansive domain of modern technology, zirconium compounds emerge as silent yet critical heroes. They propel advancements in electronics and casting luminescent wonders in optoelectronics.
For instance, zirconium oxide (ZrO2) and its derivatives, stand as keystones in electronic components. They also have optical applications thanks to their multiple properties.
This article explores the pivotal role of zirconium compounds in electronic and optoelectronic advancements. Hope that it shows their contributions and potential for future innovations in these fields.
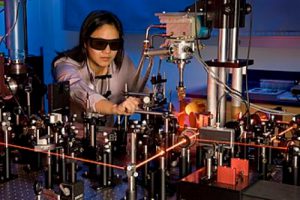
Zirconium Compounds: Types and Properties
Zirconium compounds are a diverse family of materials with an array of types.
- Zirconium oxide (ZrO2) is known for its exceptionally high melting point and remarkable stability at extreme temperatures. It is a prominent member.
- Its variants, such as yttria-stabilized zirconia (YSZ), exhibit enhanced toughness, excellent thermal insulation, and mechanical resilience. So, they find extensive use in thermal barrier coatings, dental ceramics, and solid oxide fuel cells.
- Additionally, zirconium silicate shows remarkable wear resistance and finds application in ceramics, and refractories. It is an opacifier in glazes and enamels, owing to its ability to impart opacity and brightness.
These compounds possess high-temperature resilience. They also have wear resistance to superb electrical and thermal properties. Thus, they are indispensable in a multitude of industrial applications.
Related reading: 6 Uses Of Zirconium You Mightn’t Know
Pioneering Role in Electronic Components
Zirconium compounds find their digital footprint primarily in the realm of electronic components. So, they have innovations through their diverse attributes:
- Dielectric Innovations: Zirconium oxide is renowned for its high dielectric constant. It takes center stage in electronic components and empowers the creation of high-k dielectric films. These films are integrated into capacitors and semiconductor devices. They enhance performance by facilitating efficient charge storage and transfer.
- Enhancing Transistors: In the intricate landscape of transistors, zirconium compounds contribute significantly to insulating layers, like hafnium-zirconium oxide combinations. These layers adorn metal-oxide-semiconductor field-effect transistors (MOSFETs) and ensure seamless electronic switching with reduced power consumption.
The Optoelectronic Sphere
Beyond traditional electronics, zirconium compounds are also useful in the realms of optics and light-driven technologies:
- Optical Marvels: Zirconium compounds take the spotlight in optical coatings, especially zirconium dioxide thin films. These coatings adorn lenses, photonic devices, and optical filters. They reduce glare and enhance light transmission through their anti-reflective properties. So, they can refine visual experiences and optical functionalities.
- Vibrant LED Technologies: The realm of light-emitting diodes (LEDs) witnesses zirconium compounds shaping phosphors. That’s pivotal for producing diverse colors and efficient light emission in LEDs. This contribution enriches displays and lighting technologies, infusing them with vibrant hues and energy-efficient illumination.
Charting the Course for Future Innovations
Amidst current achievements, zirconium compounds pave paths toward future breakthroughs and sustainable technological endeavors:
- Nanoscale Exploration: The ongoing exploration of nanostructured zirconium compounds aims to unlock unique properties at the nanoscale. These research and experiments propel advancements in electronic devices and optoelectronic systems by harnessing their novel attributes.
- Sustainable Technological Horizons: In a concerted effort toward sustainability, researchers endeavor to craft eco-friendly zirconium-based materials for electronics and optoelectronics. These materials aspire to minimize environmental footprints while accentuating recyclability in electronic devices.
Conclusion
In a word, zirconium compounds stand as unsung heroes. They drive the evolution of electronics and illuminate the paths of optoelectronics. Their multifaceted roles range from empowering electronic components with enhanced performance to adding brilliance to optical applications. As research advances, these compounds forge pathways toward sustainable innovations, promising a future illuminated by eco-conscious technologies and ever-evolving electronic marvels.
Stanford Advanced Materials (SAM) stands as a reliable and trusted supplier. SAM IS renowned for offering an extensive spectrum of zirconium compounds. We provide a diverse array of high-quality zirconium metal products meticulously curated to meet industry needs. Send us an inquiry if you are interested.
Reference:
[1] Optoelectronics. (2023, November 2). In Wikipedia. https://www.wikidata.org/wiki/Q193091